Malaria is a disease caused by an infection of parasites. Malaria is difficult to treat and causes significant morbidity and mortality. Treatment can be intense and difficult for the patients. According to WHO statistics in 2017, globally there were 219 million malaria patients, of which 28% died from the disease.
In many regions of the Earth in which malaria was endemic, its eradication was achieved, both for the elimination of the parasites that cause it, and for the prevention of the bite of the mosquitos that transmit it, typically from the genus Anopheles.
But the struggle against the parasite responsible for the disease remains. It is estimated that more than $ 3 billion has been invested worldwide in control programs for this disease, including funding for important research programs that attempt to discover how to maintain and control the parasite outside and inside the human body and infected animals.
Much of the knowledge gained from research in this long struggle is attributed to in-vitro studies of plasmodium, (1), with the aim of understanding their complex life cycle and potential fragility that might allow the development of therapeutic drugs and vaccines to molecular or cellular targets. The ultimate goal of this work is to prevent transmission of the disease and/or eliminate the parasite in infected organisms.
Attempts for in-vitro culture of human infecting plasmodia were published at the beginning of the 20th century. In 1911 Bass, at Tulane University (New Orleans), issued an extensive article (2) on the possibility of maintaining in vitro, about a week, the parasites such as Plasmodium Vivax, P. Malariae, P. Ovale, P. Knwlesi and P. Falciparum. The basics of these protocols was their culture in a medium composed by human blood (3), without fibrin, with citrate, at a temperature of 40º C and in an atmosphere without oxygen or with very low oxygen.
Since Bass’ publications, more than 300 papers reported results on plasmodia maintained in-vitro, as a tool of research in biology and medicine, including several that extended the basic protocol to methods to maintain Plasmodium in vitro in continuous cultivation (4). Overall, a culture protocol for Plasmodium species has been standardized, with the fundamental requirements being a glucose-rich cell culture medium, and pH within 7.2 and 7.54, sustained with HEPES or bicarbonate buffer. This medium is then supplemented with human A+ group erythrocytes, or erythrocytes of some primate species, such as chimpanzees or nocturnal monkey. As for the culture container use to grow them, it does not require anything special, except to provide a low oxygen media environment. This requires keeping traditional culture vessels inside 5% CO2 incubators at 37 OC and an atmosphere between 5% and 8% oxygen (4).
The need for low oxygen and high exogenous CO2 complicates the culture procedure because the cell culture containers must remain inside gas-controlled incubators fixed in a lab. The oldest and simplest procedure used to achieve these needs, is to introduce the cell culture devices inside candle jars (fig. 1A) or hermetic chambers injected with a mixture of gases as industrially modified air. Candle jars are hazardous for transportation and need to be incubated in a classic incubator. If the culture will not be transported, a practical old solution is to use a tri-gas incubator, with attached canisters of CO2, N and O2 (5). These tri-gas controlled hypoxic chambers are expensive and cumbersome because these require a permanently connected gas canister.
Cell culture in hypoxic atmosphere has been used in cell biology research for more than 20 years to investigate the responses of mammalian cells to oxygen deprivation. All these researchers used hypoxic chambers and incubators based on the displacement of the Oxygen by massive Nitrogen flow. However, the real environment of the cells is not the air of the incubator, it is the water-based medium were the cell are living. Although creating an atmosphere poor in oxygen will allow in time the equilibrium of the oxygen in the air into the liquid medium,
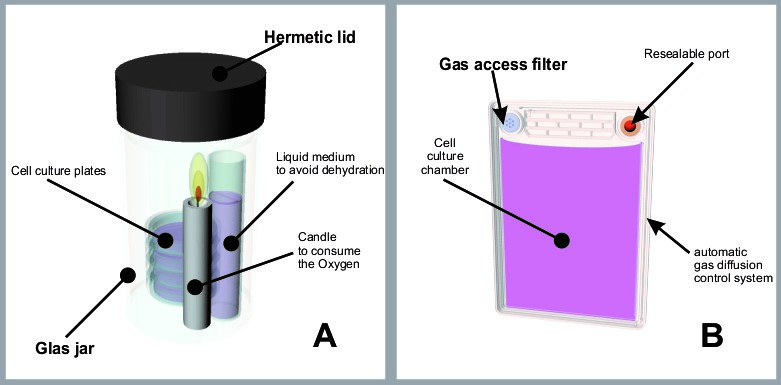
Figure 1
it is quite uncertain that the concentration of oxygen in the medium will be identical to the concentration set for the air, throughout the entire experimental period. In fact, it is well known that multiple dishes placed in an identical gas environment may have very different pO2 levels in the medium at the level of the cultured cells depending on factors such as the height of the medium column above the cells. This is why even using dedicated, so-called hypoxia chambers or tri-gas incubators set at a determined O2 gas level the actual O2 level in the culture is a complete mystery during the actual experimental cell culture period.
At the beginning of 21st century the idea of avoiding the heavy and hazardous high-pressure gas canisters began to take hold. Soon advanced cell culture devices featuring Ducted Respiratory Chamber (DRC) technology were created (6), which controlled the gas diffusion into the media with a combination of microchannels and polymer barriers. These culture devices, or bioreactors, are virtually hermetic, therefore the dissolved oxygen of the media is not replaced by direct diffusion from the atmospheric gases. When oxygen is consumed and CO2 released by the cell population; the calibrated diffusion system of the DCR automatically allows access to the medium of only a small quantity of gases in both directions, in feedback with the oxygen consumption rate and metabolism of the cell population, achieving an ideal physioxia. These bioreactors commercialized under the name of PetakaG3TM (Fig 1B) have been used with several clear advantages: first, expensive tri-gas incubators and the necessary gas canisters are not needed at all to achieve optimal low oxygen culture. Second, the oxygen concentration is controlled by cellular oxygen demand, and through intimate feedback with the cells, automatically maintains an ideal oxidative environment for all kinds of cells. Third, the bioreactors are small, low-cost, absolutely portable and robust for any purpose.
These three characteristics are enough to strongly recommend the use of Petaka G3™ for growing and transporting Plasmodium cultures. Recently, one team of researchers published the indisputable effectiveness of the Petaka G3 in Plasmodium maintenance, (7) and discuss the usefulness and advantages of these protocols compared with the other current protocols.
Culturing Plasmodium, a parasite of 1 or 2micron size, in PetakaG3™ is recommended for many reasons included reducing contamination risk. As soon as a blood infected sample is injected into the device, contamination is impaired by the permanent sterile closure provided by the self-sealing port and the 0.2-micron filter protecting the automatic gas diffusion control system. Both allow the introduction of fresh culture medium and removal of old medium, with or without cell retrieval, all in sterile conditions. In addition, the Petaka G3™ bioreactor, offers the possibility of measuring and recording the oxygen level in culture and the oxygen consumption rate of the growing parasite population, a very important area of research for drug development against malaria (7).
According to the publication of A. Géry (7), the culture protocol found to be both practical and effective, can be performed without a laminar flow system when using the Petaka G3™. This particular protocol facilitates the field operations in a way that was not previously possible:
- Use as culture medium, a slight modification of the original methods developed by Haynes and Trager, consisting in type A+ erythrocytes at 5% hematocrit, suspended in RPMI 1640 medium, supplemented with 25 mM HEPES, 25mM NaHCO3, 1 mg/L hypoxanthine, 2 mM L-glutamine and 0.5% lipid rich bovine serum albumin (BSA).
- Seed initial parasitemia set at 0.5%, in 75-cm2 in Petaka G3™ FLAT (for nonadherent cells). Containing 25 ml of culture medium–blood mixture to fill the internal chamber completely, without leaving any air space inside.
- Incubate in the room-air microbiological incubator (no CO2 incubator is needed), at 37OC for 30 days.
- Change the culture medium every 4 days, using sterile syringes and tips to remove the old medium.
- Add fresh type A+ erythrocytes to reduce the parasitemia by half, when parasitemia attains 10%.
- From the spent medium, divide the red cell pellet (2 ml) into two equal fractions, one fraction to be cryopreserved, and the other to be returned to its original device, to which 1 ml of uninfected erythrocytes may be added.
The authors, also note that with cultures in the Petaka G3™, a change only every 4 days is should be sufficient to maintain a long-term culture of Plasmodia, however in flasks and dishes a daily change is often needed.
REFERENCES
- Yotoko, K.S.C., Elisei C. 2006: Malaria parasites (Apicomplexa, Haematozoea) and their relationships with their hosts: is there an evolutionary cost for the specialization? J. Zoo. Syst. Evol. Res. 44 (4): 265
- Bass CC, Johns FM. The cultivation of Malaria Plasmodia (Plasmodium Vivax and Plasmodium Falciparum) in vitro. (1912) J Exp Med. Oct 1;16(4):567-79.
- Haynes, J. Diggs C., Hines, F., Desjardins, R.1976. Culture of human malaria parasites Plasmodium falciparum. Nature. 263, pages767–769 (1976)
- Trager, W. & Jensen, J. B. Human malaria parasites in continuous culture. Science 193, 673–675 (1976)
- Minimal tri-gas incubator. Extracted from: https://www.youtube.com/watch?v=3LWZfmC1Auk
- Gallagher M.J. and Barbera-Guillem E.(2012) GEN November 1, (Vol. 32, No. 19)
- Antoine Géry et al. (2019) Long-Term In vitro Cultivation of Plasmodium falciparum in a Novel Cell Culture Device. Am. J. Trop. Med. Hyg., 100(4), pp. 822–827.