Traditional Cryopreservation
Cryopreservation of cells is an essential tool for modern laboratory research. The ability to store biological material indefinitely and thaw it in a way that reliably maintains viability is invaluable. Under normal conditions cells die during freezing from the mechanical trauma of uncontrolled ice formation and the chemical damage from changing solute concentration, (for an excellent review, read Wowk, 2007) 1. The processes of controlling ice crystal formation during the freezing process is complex. Critical elements of the equilibrium cryopreservation process include: depressing the freezing point, managing osmolarity, minimizing cryoprotectant toxicity, allowing for timely cellular dehydration, etc. Because there are so many critical factors, slow-cooled cryo-freezing is complicated, requires a lot of time and can be unreliable.
The typical steps in cell cryopreservation involves removing culture medium, releasing adherent cells, transferring to a centrifuge tube, concentrating by centrifugation, pouring off excess medium, re-suspending in freezing medium containing DMSO, aliquoting into cryovials, gradually cooling to allow for dehydration of the cells and concentration of the cryoprotectant inside the cell compartment, then finally exposure to liquid nitrogen, (see Meneghel, et al.) 2 Once frozen, cells can be maintained in a biologically inanimate state until needed.
Just as slow, controlled cooling is critical in the traditional freezing process, rapid warming is critical to the thawing process. Although it seems counterintuitive, as the sample warms from below -120°C towards -0°C, fresh ice nucleation and ice crystal propagation may occur, leading to cell membrane damage and dead cells. Because of this phenomenon, it is commonly written that cell frozen in cryovials should be thawed in less than one minute, but everyone knows that cryovials with 1-2ml of frozen medium can often take two or three minutes to become ice-free when warming in a 37°C water bath. Many labs still get acceptable results with this primitive and slow warming method, but in any case, cryo-frozen samples should be thawed as quickly as possible for ideal cellular recovery.
Vitrification
An alternative to the traditional slow cooling method is to vitrify the sample, which means freezing without inducing ice crystal formation3. When samples are vitrified in the absence of ice there can be no membrane damage from growing ice crystals. Vitrification also eliminates damage from solute and osmotic effects, and eliminates cryoprotectant toxicity when performed at a low cryoprotectant concentration or with no cryoprotectant at all. The physics behind vitrification are complex, especially for large tissues and organs, (see lecture by Dr. Wowk : https://www.youtube.com/watch?v=9otFo1HI5U0 ), but for low mass samples that can be frozen and thawed extremely rapidly, (in microseconds) a form of kinetic vitrification is known to preserve cell samples with little or no cryoprotectant. This technique has been described many times in fertilization methods but rarely for cells in culture. The idea is simple, if you can freeze super rapidly and thaw super rapidly, cells in a very thin layer and in a very small volume will vitrify safely, without excess ice formation, and with little or no cryoprotectant required. This would be an ideal way to cryo-process large numbers of cells, but current container technology prevents this.
Why are Cryo Vials a Bad Choice?
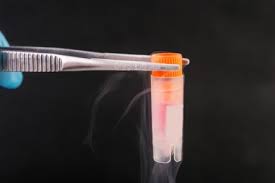
For decades, the state of-the-art container for laboratory use has been the standard 1ml or 2ml polypropylene cryovial4. In order to withstand the stress of liquid nitrogen exposure, cryovials are constructed with very thick walls and heavy threads compared to most microtubes, but this design means that heat transfer during freezing and thawing is extremely slow. In addition, the cylindrical inner shape forces the liquid sample into one of the least favorable configurations for freezing. Liquid in a cryovial has an extremely low surface-to-volume ratio, so neither freezing nor thawing can be achieved rapidly using cryovials.
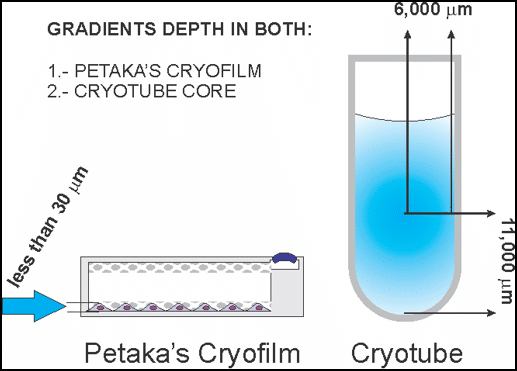
As seen in the figure (right), rapid freezing is not possible in cryovials since the freezing front advances slowly through the thick column of liquid from the outside inward. Likewise, the sample thaws very slowly as the liquid front advances from the outside edge inward, allowing plenty of opportunity for damage from ice crystals. This is why the classical slow cooling method is mandatory when using cryovials and why thawing takes so long. It is clear that the cryovial is not well suited to rapid freezing or thawing. Moreover, requiring thawing in a water bath means that threads, o-rings and the body of the vial is typically covered in microbial contamination immediately prior to going into the laminar flow hood. This is no doubt the cause of innumerable contamination events when thawing critical banked specimens.
The Ideal Cryo-container

The ideal cryo-container would serve as both the culture container and cryo-container, allowing for cryoprocessing and long-term storage without trypsinization, centrifugation, or open transfers of cells. Obviously traditional flasks, bags, dishes and plates are unsuitable since they are too large, would not remain sterile, and are not rugged enough to be cryofrozen. The ideal device would likewise have extremely thin walls (<1mm) and would maintain the attached cells with the maximum surface-to-volume ratio, allowing extremely rapid freezing and thawing. Finally, the ideal cryo-container would be functionally closed to maintain sterility as samples are transferred into and out of freezers and LN2 dewars.
The Ideal Freezing Process
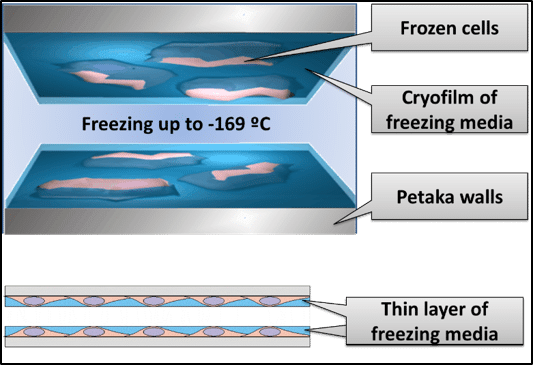
The ideal freezing protocol allows cells to be cryopreserved while still attached to the surface of the original cell culture container. This would eliminate harsh trypsin treatment, maintain the cells in their native configuration with all of the mechanical protection that attachment brings, and eliminate transfer and centrifugation steps which add contamination risk. In this ideal process, the user briefly exposes the attached cells to the cryopreservation solution, aspirates excess cryoprotectant (leaving only 1ml of liquid for up to 15 million cells), then rapidly flash freezes cells directly. Flash freezing a thin-film of freezing medium and cells (<30um thick) in a process described as “kinetic vitrification”, provides all the benefits5 of low ice crystal formation, no cellular dehydration, and low cryoprotectant toxicity. Once flash frozen, samples may be maintained indefinitely in the LN2 vapor phase.
The Ideal Thawing Process?
The ideal thawing process would entail removing the frozen container with attached cells and thawing nearly instantly by adding a large volume of warm culture medium. This method also eliminates slow thawing of samples in dirty waterbaths to provide the cleanest and most hygienic recovery process possible. This process also eliminates transfers from cryovials into new cell culture devices, and immediately provides the attached cells, in their original configuration, metabolizing, and growing within minutes, eliminating the re-attachment and recovery time typical when recovering adherent cells from cryo storage.
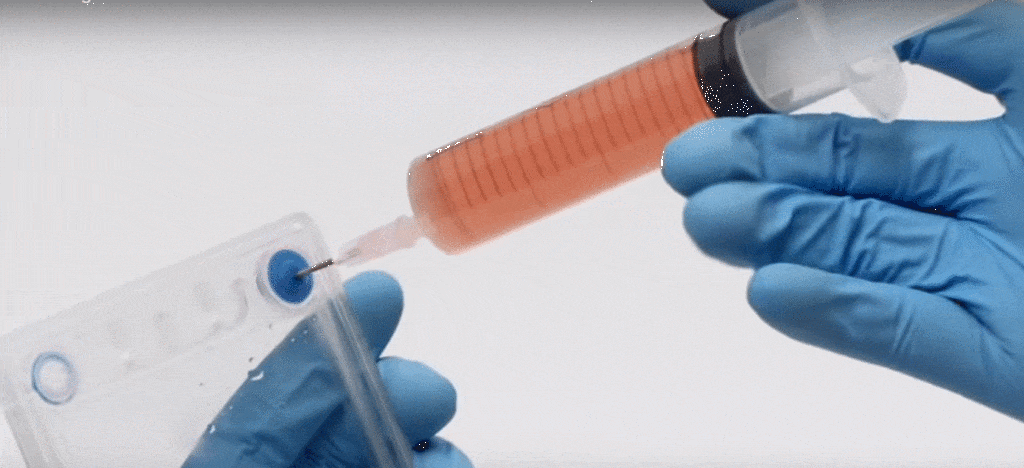
The Petaka G3™ Solution
The theoretical device and protocols described above are no longer just wishful thinking. The Petaka G3™ perfectly supports the idealized thin-film, freeze-thaw process described above. The Petaka G3™ is a rugged, sterile, single-use cell culture device that allows for a highly-efficient thin-film procedure for rapid freezing and thawing. Since the cells grown on the Petaka surface can be frozen in place, there is no longer a need to trypsinize, centrifuge and resuspend the cells in freezing medium. Since the cells in thin-film freeze rapidly there is no damage from osmotic changes and excessive cellular dehydration. And since the cells are thawed by filling the Petaka G3™ with warm medium, the attached cells thaw practically instantaneously; there simply is no time for uncontrolled ice crystal formation to mechanically damage cells. In the process using the Petaka G3™ cells are never centrifuged or transferred during freezing or thawing and no dirty water baths are required. Finally, cells are frozen in such a small volume of freezing medium, the trace quantity of cryoprotectant is typically diluted to below 0.4% in the fresh culture, so no post-thaw rinse is required.
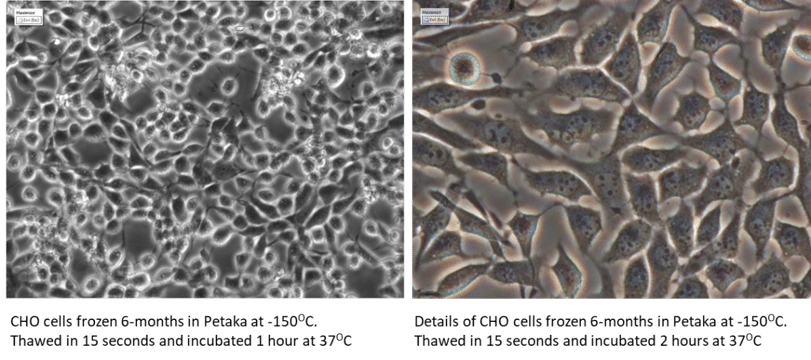
The Petaka G3™ Cryopreservation Freezing Protocol
- Aspirate growth medium from Petaka G3™ with attached cells
- Add freezing medium to Petaka G3™, cover all surfaces and leave for 30 seconds
- Aspirate freezing medium, leaving ~1ml of freezing medium
- Immediately freeze Petaka G3™ with cells attached (freeze instantly)
The Petaka G3™ Cryopreservation Thawing Protocol
- Remove selected Petaka G3™ from cryo storage
- Fill with warmed, complete cell culture medium (thaw instantly), do not rinse.
- Place Petaka G3™ with attached cells in incubator in horizontal position
The Petaka G3™
The Petaka G3™ is used across the globe to grow cells under physioxic conditions, to ship live cells at ambient temperature for 7-10 days, to centrifuge and transfect cells, to do reliable real-time benchtop microscopy, and to monitor oxygen levels in culture in real time. However, of all these unique attributes, thin-film cryopreservation in the Petaka G3™ may be the most groundbreaking because nothing like this protocol has been described before. No other technology exists that allows cryopreserved cells to be stored in the lab or shipped frozen to customers while still attached and in their native configuration. No other technology allows for fresh, healthy “assay-ready” cell recovery simply by adding warmed medium. Additionally, because the Petaka G3™ is so thin and compact, cell densities achieved in standard cryoboxes is comparable to what can be achieved with a standard 81 cryovial configuration.
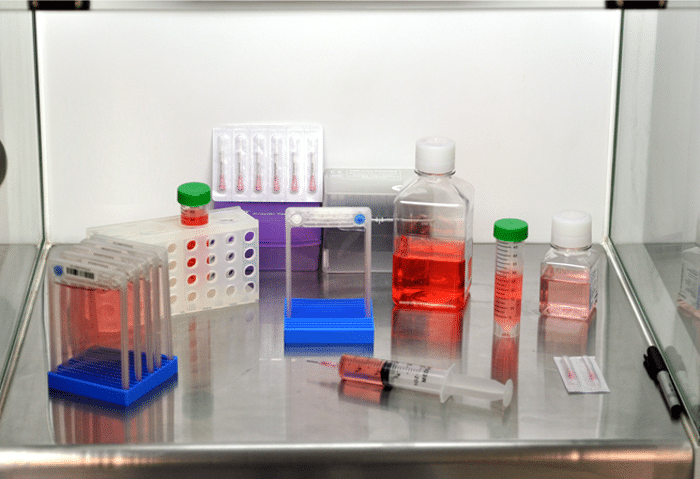
The shift from the traditional and complex, slow-cooled cryopreservation methods to the rapid, thin-film method could represent a major advance in routine cell culture management and biobanking that is underappreciated, and this method is only possible in the Petaka G3™.
REFERENCES
- Brian Wowk. Cryonics, third quarter, 2007, 1-5.
- Meneghel, et al. PLOS One 2019, 14(5): e0217304
- Fahy and Wowk. Methods Mol Biol 2015; 1257:21-82.
- Charles Hunt. Transfus Med Hemother 2019;46:134-149.
- Von Bomhard, et al. PLOS One 2016, 11(2): e0149660