Eukaryotic cell culture was developed with the use of open containers such as Petri dishes and flat bottles. Many years of experience and frequent contamination with airborne germs led to the addition of closures for the culture vessels, a lid in the case of the plates or a cap in the case of the bottles. Eukaryotic cells are grown at 37 °C in an aqueous medium equipped with all the necessary molecules to generate a sufficient number of cells. Among the molecules contained in the medium are two important dissolved gases, oxygen (O2) from the atmosphere and carbon dioxide (CO2), as a product of cellular aerobic respiration.
A reliable cell culture medium must maintain a relatively neutral pH during the entire culture period, which is commonly achieved by adding a bicarbonate buffer to the medium. The CO2 naturally released by the cells can easily maintain the balance of the bicarbonate buffer, but only if the CO2 produced does not escape from the medium into the open atmosphere. When culturing cells in common plates, dishes, flasks and bags, the CO2 rapidly escapes the medium resulting in high pH levels incompatible with a healthy culture.
CO2 at 37 °C is very soluble in water, so when CO2 is added to the incubator atmosphere (5% is common), it will rapidly dissolve in the culture medium. However, the concentration of CO2 in the incubator cannot exceed a certain level either, because too much CO2 will acidify the medium with serious negative consequences for the cells. Incubators for the cultivation of cells featuring precise control of the temperature and the CO2 concentration have been available for many years, but these incubator systems require the continual maintenance of external CO2 canisters.
Oxygen in the atmosphere today (about 21%), will readily dissolve in the medium, until saturation. This equilibrium result with a partial pressure of oxygen (pO2) in the medium of about 150 mmHg, however, this concentration of dissolved oxygen in the medium is not physiological for cells. In fact, cell cultures maintained in the right oxygen tension is achieved by displacing the normal oxygen from its atmosphere by flushing with nitrogen gas. This partial depletion of oxygen from the atmosphere makes it possible to control the oxygen concentrations in the medium, thus allowing a more physiological culture condition to be achieved. This also requires the user to maintain heavy and bulky external Nitrogen containers. After careful analysis and consideration, however, intelligent operators may appreciate that there are more elegant ways to achieve this state without resorting to crude flushing of gasses every time the incubator door is opened.
The amount of dissolved gases in the culture medium is governed by three basic laws of physics: Henry’s law, Graham’s law, and Fick’s law. The consideration of these laws when designing an instrument to grow cells allows the achievement of the right gaseous conditions of the medium, necessary to grow a large majority of the cell types under perfectly physiological conditions. The variables involved in these laws are seven: First, the surface area of interface between the gas environment and the media environment, limits gas diffusion across the interface. Therefore, plates, dishes and flasks with large media interface surfaces allow high gas transfer rates. Second, the difference in concentration of gas at both sides of the interface; the gas will diffuse from higher to lower gas concentration. Third, the diffusion index of the gas in the medium, or capacity of the gas to progress through the medium. Fourth. The thickness or depth of the medium; the deeper it is the longer the time of diffusion, and slower the flow of the molecules. Fifth, the solubility of the gas in the media; as higher gas solubility means more gas will be retained in the medium. Sixth, the temperature, which influences the partial pressure of the gases, their solubility, and diffusion coefficients. Finally, Seventh, the time of contact between both environments, so the longer the time of diffusion the more gas will be transferred.
So, by creatively controlling these seven variables within the cell culture container itself, it is possible to manage the adjustment of gas concentrations within the cell culture device itself. This frees the operator from any external gas input in order to achieve physiologic culture, thus rendering unnecessary external tanks of high-pressure gases, environmental control apparatuses, and complex feedback regulating devices. The design of a closed cell culture container with the appropriate interface surface between the medium and the atmosphere will simply, reliably and elegantly restrict the exchange rate of each gas between both environments (air and medium), and therefore, the specific concentration of the target gas in the specific medium.
Such an elegant and passive gas control system has already been created and cleverly integrated into a line of advanced cell culture devices that provide excellent, passive control of dissolved gasses in the culture medium. The restriction of gas diffusion through a calibrated microchannel, carefully adjusted to the desired diffusion rate of the gases in the culture medium, gently and naturally reduces the pO2 in the medium while simultaneously increasing the pCO2. This constitutes one of the foundations of the Ducted Respiratory Chambers (DRC), or Snorkel chambers for cell culture. This is an example of the technological application of Fick’s laws (Fig. 1 A).
By restricting gas diffusion through an integrated air microchannel into a hermetic culture device, as the cells consume oxygen, the pO2 will decrease, and since the concentration of oxygen in the atmosphere remains constant, the oxygen in the atmosphere will then diffuse in the medium until the two partial pressures are balanced. The result is the culture will quickly reach and maintain physiologic pO2 levels (fig. 1B). Similarly, as CO2 builds up in the culture medium, excess gas will diffuse from the medium to the atmosphere through the microchannel, equilibrating at a dissolved CO2 level ideal to maintain optimum pH in the medium.
The technological solution applied by the designer of the Petaka G3™, to restrict the communication between the space of the culture medium and the atmospheric space, has come from the specific application of Fick’s law. Because a capillary of suitable section and length works like a differential pressure valve, according to the equation:

Where:
J = Gas flow
D = Oxygen diffusion coefficient in the medium
A = Capillary section area
L = Capillary length.
PO2atm = Partial oxygen pressure in the incubator atmosphere.
PO2med = Partial pressure of oxygen in the culture medium.
Therefore, the length of the capillary acts as a progressive restrictor, imposing an open/closed condition depending on the oxygen differential (PO2atm-PO2med), divided by the length of the capillary. The practical effect of this technology means that the net flow of oxygen into the culture medium rises as the cell population grows, and it is reduced as the cell population reduces its consumption of oxygen. In short, the microchannel-based gas control system featured in the Petaka G3™ auto-regulates the availability of critical gasses to provide more gas as cells require more and less when cells require less. This gas auto-regulation feature is unique to the Petaka design.
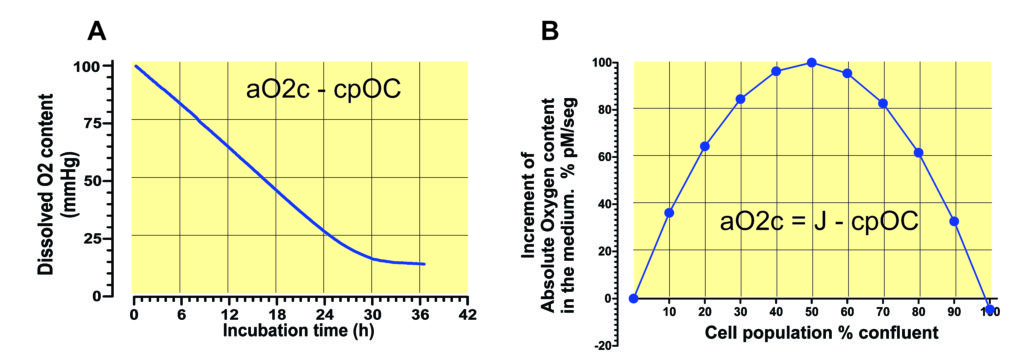
Eventually, the oxygen inflow halts when the cell population stops consuming significant amounts of oxygen. However, the increase in oxygen concentration in the culture medium follows a different equation (Fig. 2), because the influence of the size of the cell population, its oxygen consumption will compete with the difference in partial pressure between the atmosphere and the culture medium, so that the instantaneous increase in oxygen in the culture medium is equal to the difference between the oxygen inflow and the oxygen consumption due to the cell population size (Fig. 2, CPC).
One major advantage of this passive, auto-regulating system is that as the cells become confluent and the oxygen content of the medium has reached its minimum if the cells do not continue to consume, the flow of O2 into the chamber and into the medium resumes, eventually providing enough oxygen to promote the proliferation of the remaining active cells. If a majority of cells do not receive enough oxygen, most of them enter into a kind of pausing or dormancy, with minimized metabolism. That, pausing state will once again be interrupted by a critical level of oxygen availability, and a sort of cycle is established of cell activity/cell dormancy, and concomitant physioxia 1] (Fig. 3).
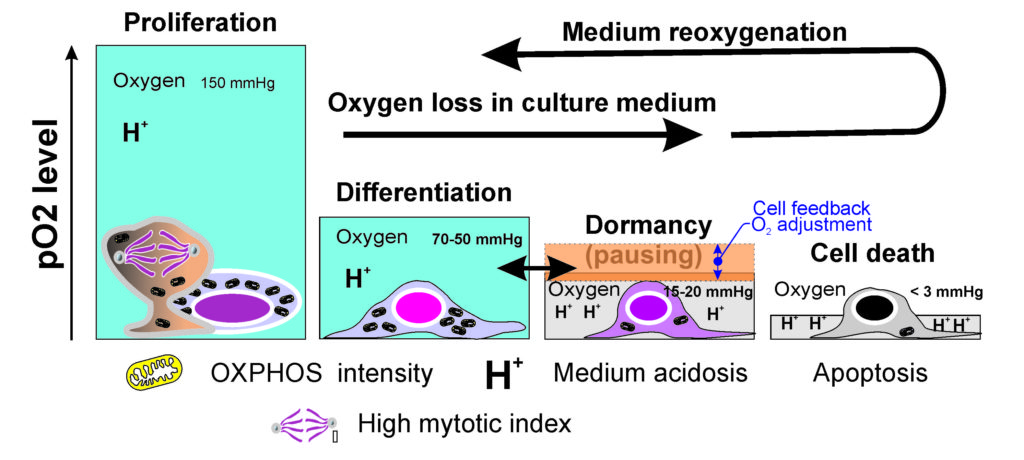
These principals are important if one is to understand how the Petaka G3 cell culture technology passively provides sensitive and automatically regulation of physiologic oxygen levels to cells in culture, while simultaneously retaining sufficient CO2 to maintain the pH of culture medium. This is how the Petaka G3 cell culture cassette allows for ideal gas control without the need for tri-gas incubators, glove boxes, hypoxic chambers or environmental control boxes. All the one needs for excellent physiologic cell culture is already contained in the plastic disposable and functions automatically, with no operator input required.
[1] Physioxia: Oxygen concentration in which the cells live in the natural tissues.